Polarization: Difference between revisions
Lwinalski3 (talk | contribs) No edit summary |
No edit summary |
||
(90 intermediate revisions by 7 users not shown) | |||
Line 1: | Line 1: | ||
Edited By: siyer300 - Samyukta Iyer, Spring 2023 | |||
Naeha Nayar - Spring 2024 | |||
In essence, polarization describes how charged objects interact with each other in terms of the separation of charges within such objects. This article refers to electric polarization. For magnetic polarization, check [[Magnetic Dipole]] | |||
<br> | |||
== Definitions, at a Glance== | |||
Polarization is defined theoretically [http://www.physicsclassroom.com/class/estatics/Lesson-1/Polarization defined] as "the process of separating opposite charges within an object" by the application of an external electric field. Polarization is the separation of the center of positive charge and the center of negative charge in a material. | |||
Let us first consider the case of an atom (an electric dipole) being exposed to an external electric field. | |||
Essentially, exposure of an atom to an external electric field causes the electron cloud of an atom to surround the positively charged nucleus of the atom opposite the direction of the electric field, causing one side of the atom to be positively charged and one side to be negatively charged. | |||
As you know, negative and positive will always repel each other. So when the positive and negative charges within an object have become separated, the object is said to be polarized. | |||
Due to the physical separation of its positive and negative charges, a polarized object can behave like a dipole, so long as the charges remain separated. Once the electric field causing the polarization is removed and the positive and negative charges are no longer separated, the dipole disappears. | |||
===Diagram=== | |||
This is also explained succinctly in the diagram below: | |||
Because of this phenomenon, polarization is said to create [https://www.youtube.com/watch?v=5O0yWvQhWkU induced dipoles]. | |||
[[File:lastdrawing.jpg|lastdrawing|300px|Image: 300 pixels]] | |||
===Polarizability=== | |||
Given the phenomenon of polarization, we can then define polarizability as the ease with which the charges in an object can be separated. It measures the tendency of matter to acquire an electric dipole when it is affected by an electric field. Polarizability is a constant value, represented by the Greek character alpha, that is inherent and unique to each material and is commonly determined experimentally. It is measured in units of volume such as cubic meters. Polarizability influences a material's ability to store electrical energy when subjected to an electric field, which is important in dielectric materials used in capacitors and insulators. Molecules with more loosely held electrons or that have larger electron clouds tend to have higher polarizabilities. | |||
Polarizability affects the strength of van der Waals forces and London dispersion forces between molecules, which influence properties such as boiling points, melting points, and solubility. Polarizability also determines how a material interacts with light, affecting the ability to scatter or absorb light. | |||
===Dipole Moment, in General=== | |||
The amount of polarization an object experiences, also known as the dipole moment, is equal to the polarizability of the object multiplied by the magnitude of the applied electric field. | |||
<math> µ = α * E </math> | |||
Where µ represents the dipole moment, α represents the polarizability of the given object, and E represents the applied electric field's magnitude | |||
** note that E and mu have a direct relationship so as the electric field's magnitude increases, the dipole moment will increase as well. | |||
===Polarization in Insulators vs. Conductors=== | |||
Insulators: | |||
An insulator is a material through which mobile charges cannot flow, and a conductor is a material through which mobile charges can flow. | |||
In an insulator, the separation of charge simply causes the electrons of the object being polarized to position themselves on the outer surfaces of the object. | |||
It contributes to the material's dielectric properties, affecting its ability to store electrical energy when it comes into contact with an electric field. | |||
Polarization can lead to the formation of surface charges at the interfaces between the insulator and its surroundings, which can influence the material's behavior in various applications. | |||
In ferroelectric materials, polarization can be permanent and exhibit hysteresis behavior, meaning that the material retains its polarization even when the external electric field is removed. | |||
[[File:PVC pipe Example.jpg|PVC_pipe_Example|300px|Image: 300 pixels]] | |||
Conductors: | |||
Conductors are materials characterized by their high electrical conductivity, meaning that they have free electrons that can move easily through the material in response to an applied electric field. | |||
In conductors, the electron field induces a flow within the conductor of electrons from the heavily negatively charged side of the object to the heavily positively charged side of the object. When a conductor comes into contact with an external electric field, the free electrons redistribute themselves almost instantaneously to cancel out the electric field within the conductor. This redistribution of charges leads to an equilibrium state where the net electric field inside the conductor is zero. | |||
[[File:GOLD COATED STAINLESS STEEL BLOCK - NARA - 17474918.jpg|GOLD_COATED_STAINLESS_STEEL_BLOCK_-_NARA_-_17474918|300px|Image: 300 pixels]] | |||
====Clarification==== | |||
It is important to note that the process of polarization in and of itself does not induce charging. Polarization is the redistribution of charges throughout an object; a polarized neutral object is still a neutral object regardless of whether it is an insulator or conductor. | |||
Simple schematic of a polarized molecule, or a dipole | |||
[[File:dipole1.jpg]] | |||
==The Main Idea== | ==The Main Idea== | ||
At | At the atomic level, the application of external charges causes the subatomic particles, namely, positively-charged protons and negatively-charged electrons, to reorient with respect to the applied charge. | ||
The external application of a positive charge on the left side of a molecule will result in the electrons of the molecule moving to the left, as they are attracted to the positive charge, and the protons stay to the right, away from the applied charge, as they repel against the positive charge. | |||
[[File:Steps of polarization.jpg|thumb|]] | |||
Similarly, the external application of a negative charge on the right side of a molecule will result in the protons of the molecule staying to the right, close to the applied charge, as they are attracted to the negative charge, and the electrons of the molecule move to the left, away from the applied charge, as they are repelled by the negative charge. (See [http://www.physicsclassroom.com/class/estatics/Lesson-1/Charge-Interactions Charge Interaction]) | |||
<br> | |||
===Connection between Electric Fields and Induced Dipoles=== | |||
<br> These externally applied charges, as described earlier, are electric fields. Positive electric fields point outwards, away from the center of the field, also known as its source. Negative charges, on the other hand, create inward-pointing electric fields that point toward the source of the field. The external application of a positive electric field to an object will create an outward field, resulting in the movement of the electrons of a molecule closer to the external positive charge. | |||
The positively-charged nucleus, while it does repel against the external positive charge, cannot move around the molecule however as that would change the molecule itself. With the charges physically separated in space, an induced dipole forms. When the external positive charge is removed, the induced dipole disappears and the object is once again neutral. | |||
On the other hand, the external application of a negative electric field to an object will create an inward field, resulting in the movement of the electrons of a molecule farther away from the external negative charge. The positively-charged nucleus will be attracted to the negative charge, but as described before, because it cannot move around the molecule, will not be pulled closer to the negative charge. | |||
===Permanent vs Induced Dipoles=== | |||
Both of these instances describe scenarios drastically different from permanent dipoles, in which the positive and negative charges are always physically separated. Polarization and the resulting creation of induced dipoles help explain the seemingly magical attraction between charged objects and neutral objects. | |||
[[File:Permanent vs induced.jpg|thumb|]] | |||
A charged object produces an electric field that, when brought near neutral objects, generates induced dipoles. The protons and electrons reorient themselves in the presence of the charged object's electric field and attractive forces can be observed between the two objects. | |||
[[File:1wikibookpic.jpg]] | [[File:1wikibookpic.jpg]] | ||
Polarization in | ===Polarization in Insulators=== | ||
Insulators are materials in which the electrons are tightly bound to the atoms, preventing the movement of charged particles throughout the material. As a result, electricity, which is the movement of charges, is unable to flow through the object and it is said to "insulate" one object from another. Common examples of insulators include wood, rubber, paper, and glass. | |||
Insulators have very low polarizability constants. I.e., insulators do not polarize easily. In the presence of an externally applied electric field, the electrons in an atom shift positions slightly, but are unable to move and become separated from the protons. At most, the electrons can shift one atomic diameter or 1x10^-10 meters, but they ultimately remain attached to the atoms. | |||
This results in the induced polarization of the individual molecules inside the insulator, as the applied electric field has caused the normally neutral object to become polarized, as opposed to entire charges moving across the material independent of their molecules (as would occur in a conductor). Within each of the individual molecules making up the insulator, dipoles have formed, but the molecules themselves have not moved. This is commonly described as the "pill" model, where each now-polarized molecule resembles a pill, with one side being positively charged and the other being negatively charged. | |||
The magnitude of the induced polarization is dependent upon the strength of the applied electric field. The stronger the applied electric field, the greater effect it has on the insulator. | |||
Although the molecules are not shifting, induced polarization still creates a large effect as there are many molecules to be polarized and therefore, many induced dipoles to form. In other words, the effect of the induced polarization in the insulator is felt due to the sheer quantity of slightly polarized molecules in the entire object. The separation of the positive and negative charges (polarization) is proportional to the strength of the external electric field. If the applied electric field is large enough, the induced dipoles will generate their own electric field as illustrated below. | |||
[[File:2wikibookpic.jpg]] | [[File:2wikibookpic.jpg]] | ||
Polarization in Conductors | ===Polarization in Conductors=== | ||
Conductors are materials in which charged particles are able to flow freely, unlike insulators in which charged particles are tightly bound to the atoms. | |||
Charged particles within conductors are able to move great distances, and their movement is also the fundamental concept enabling electricity. Common examples of conductors include silver, gold, salt water, concrete, and aluminum. | |||
As a result of the unrestricted freedom of charged particles, polarization in conductors differs from polarization in insulators. While the electrons in an insulator are capable of reorienting themselves, the electrons in conductors can move great distances, spreading across the entire surface in response to the application of external charge. | |||
The mobile charges can even accumulate on the outer surface of a conductor! A good example of this is Faraday's cage. A Faraday cage is essentially a hollow conductor which allows charges to accumulate on the outer surface of the cage. | |||
[[File:Faraday cage at US Bureau of Standards 1925.jpg|Faraday_cage_at_US_Bureau_of_Standards_1925|200px|Image: 200 pixels]] | |||
After an electric field is applied to a Faraday cage, the charges on the outer surface cancel out with the charges on the inside of the cage, resulting in an equilibrium state for the cage. | |||
[[File:Faraday cage.gif|Faraday_cage]] | |||
====Conductors in Equilibrium and Drift Speed==== | |||
The process of polarization may cause the mobile charges to reorient on the surface in such a way that the net electric field goes to zero as the electric field on the surface cancels out the applied electric field. When this happens, the object is said to be in equilibrium and the electrons are no longer capable of moving through the object. | |||
The speed with which the mobile charges move due to an applied electric field is known formally as drift speed. The drift speed is equal to the net electric field at the location of the charge multiplied by the mobility of the mobile charges. As made evident by this equation, when the object is in equilibrium, the charges stop moving. | |||
[[File:3wikibookpic.jpg]] | [[File:3wikibookpic.jpg]] | ||
===Static Electricity=== | |||
Static electricity is energy that builds up due to the interaction between charged objects. Simply put, [https://www.youtube.com/watch?v=W1KEgBdatN8 static electricity] is an imbalance between positive and negative charges. Because objects are polarizable, whether completely (conductors), or incompletely (insulators), when charged objects come into contact with each other, the electrons and protons within the object reorient or shift. As illustrated above, opposite charges attract, meaning negatively charged objects will experience an attractive force towards positively charged objects. On the other hand, similarly charged species will repel each other. When similarly charged objects are brought into contact with one another, the mobile particles will attempt to escape as quickly as possible. This rapid movement of similarly charged particles is known as static shock. Homeowners frequently experience static shock as they walk across a bedroom carpet and build up electrons on their bodies. When they reach for a doorknob or light switch, they experience static shock as the electrons quickly "escape," or discharge. | |||
===A Mathematical Model=== | ===A Mathematical Model=== | ||
Useful formulas for calculating polarization and its effects: | |||
Electric Force: <math>\vec{F} = q\vec{E}</math> Where "F" is the electric force, "q" is the charge, and "E" is the electric field. | '''Electric Force:''' <math>\vec{F} = q\vec{E}</math> Where "F" is the applied electric force, "q" is the charge of the object being observed, and "E" is the electric field of the object. | ||
Dipole Moment: <math>\vec{P} = \alpha \vec{E}</math> Where "P" is the dipole moment, alpha is the polarizability (different for every material), and "E" is the applied electric field. | '''Dipole Moment:''' <math>\vec{P} = \alpha \vec{E}</math> Where "P" is the dipole moment of the object being observed, alpha is the polarizability constant (different for every material) of the object, and "E" is the applied electric field. | ||
Drift Speed: <math>\vec{v} = \mu E_{net}</math> Where "v" is the drift speed, mu is the mobility of the charge, and "Enet" is the magnitude of the net electric field. | '''Drift Speed:''' <math>\vec{v} = \mu E_{net}</math> Where "v" is the drift speed of the charged particle, mu is the mobility of the charge, and "Enet" is the magnitude of the net electric field. | ||
===A Computational Model=== | |||
Use the link below to access a computational model of polarization! | |||
https://www.glowscript.org/#/user/samyukta/folder/MyPrograms/program/wiki | |||
==Examples== | ==Examples== | ||
Line 32: | Line 147: | ||
Determine if the statements below are True or False: | Determine if the statements below are True or False: | ||
1. | 1. Charged particles can flow freely within conductors. | ||
<div class="toccolours mw-collapsible mw-collapsed"> | |||
===Click for Solution=== | |||
<div class="mw-collapsible-content"> | |||
True. There are mobile charges in conductors. Insulators do not have mobile charges as the electrons are bound tightly to the atoms. | |||
Here is a diagram illustrating how charges move in conductors. | |||
[[File:Voltage source with metallic conductor-sv.svg|Voltage_source_with_metallic_conductor-sv]] | |||
By contrast, here is a diagram showing how the electrons are bound securely and therefore cannot move around between atoms when in an insulator. | |||
[[File:Covalent.png|Covalent]] | |||
</div> | |||
</div> | |||
4. True. | <br> | ||
2. The net electric field is equal to 0 when both insulators and conductors are in equilibrium. | |||
<div class="toccolours mw-collapsible mw-collapsed"> | |||
===Click for Solution=== | |||
<div class="mw-collapsible-content"> | |||
False. The net electric field is 0 only when conductors are in equilibrium. Insulators are not able to reach equilibrium. | |||
</div> | |||
</div> | |||
<br> | |||
3. Excess charges become localized on the surface of insulators, but not on the surface of conductors. | |||
<div class="toccolours mw-collapsible mw-collapsed"> | |||
===Click for Solution=== | |||
<div class="mw-collapsible-content"> | |||
False. The excess charges within conductors become localized on the surface of conductors. In insulators, the excess charges are anywhere: either on the surface or inside of the material. | |||
[[File:Conductor vs insulator.jpg|thumb|]] | |||
</div> | |||
</div> | |||
<br> | |||
4. The average drift speed of a mobile charge is proportional to the magnitude of the net electric field of the material. | |||
<div class="toccolours mw-collapsible mw-collapsed"> | |||
===Click for Solution=== | |||
<div class="mw-collapsible-content"> | |||
True. The formula for drift speed - <math>\vec{v} = \mu E_{net}</math> - shows a clear proportionality between the mobility of the charge and the magnitude of the net electric field at the location of the mobile charge. Essentially, if the drift speed of a mobile charge increases, the magnitude of the net electric field of the material will also increase, and vice versa. Similarly, if the drift speed of a mobile charge decreases, the net electric field of the material will also decrease, and vice versa. | |||
[[File:Drift speed.jpg|thumb|]] | |||
</div> | |||
</div> | |||
===Middling=== | ===Middling=== | ||
Does a negatively charged rod cause | Does a negatively charged rod cause a neutral metal sphere to polarize? If so, show the polarization of the neutral metal sphere, describe the electric field (its magnitude and direction), and electric force caused by the negatively charged rod displayed below. | ||
[[File:4wikibookpic.jpg]] | <br>[[File:4wikibookpic.jpg]] | ||
<div class="toccolours mw-collapsible mw-collapsed"> | |||
===Click for Solution=== | |||
<div class="mw-collapsible-content"> | |||
The negative electric field points towards the negatively charged rod. The electric force is also pointed towards the charged rod. Thus, the negative mobile charges are pushed to the surface of the far right side of the sphere, away from the negatively charged rod, and the positive charges remain on the left, close to the negatively charged rod. This polarization creates a large dipole which has a zero net electric field inside the sphere. | |||
<br>[[File:5wikibookpic.jpg]] | |||
</div> | |||
</div> | |||
===Difficult=== | ===Difficult=== | ||
Find and show the polarization | Find and show the polarization of Block B and Sphere C if Sphere A is a plastic sphere with a positive charge. Block B is neutral metal block while Sphere C is plastic sphere. | ||
[[File:6wikibookpic.jpg]] | [[File:6wikibookpic.jpg]] | ||
The positive charge | <div class="toccolours mw-collapsible mw-collapsed"> | ||
[[File:7wikibookpic.jpg]] | ===Click for Solution=== | ||
<div class="mw-collapsible-content"> | |||
The positive charge on Sphere A creates an electric force which repels the positive mobile charges on the block away from the positive charge while attracting negative surface charges on the block. Due to the electric force, the block polarizes in the manner illustrated below: negative charges move to the left, positive charges stay to the right. The positive surface charges on the block near sphere C cause induced polarization, forming induced dipoles within the sphere, as Sphere C is an insulator. If Sphere C were a conductor, the sphere would polarize on the outer surface, but as it stands, a mere reorientation takes places. The negative charges of Sphere C orient close to the positive surface charges of Block B. | |||
<br>[[File:7wikibookpic.jpg]] | |||
</div> | |||
</div> | |||
==Connectedness== | ==Connectedness== | ||
How is this topic connected to something that you are interested in? | |||
<br> | |||
Polarization helps explain the mystical, yet fascinating, phenomena of attraction between neutral and charged objects. Polarization is evident in our everyday lives in many ways. For example, the build up of static charge on your socks as you walk across the carpet and the shock you feel when you touch a door handle as your body discharges. As with the entire field of physics, polarization helps explain the science behind many of the phenomena we experience daily that go unnoticed. | |||
I am a computer engineering major. Honestly, I am not sure yet what Computer Engineering is all about. However, I am aware | |||
The concept of polarization itself has many industrial applications. It is seen in 3D Glasses, Infrared spectroscopy, polarized sunglasses, FM radios, and even laptop screens. There are many, many industrial applications. | [[File:Balloons (static electricity).png|Balloons_(static_electricity)]] | ||
<br> | |||
How is it connected to your major? | |||
I am a computer engineering major. Honestly, I am not sure yet what Computer Engineering is all about. However, I am aware that polarization is an important concept in electrical engineering. Polarization of light waves seems to be more connected to my major. | |||
Edit by Jerrin: As an electrical engineer, polarization is important in understanding electron flow in wires and other conductors and the effect of electric fields of charges on external power sources such as in the hardware of a computer or other electronics. | |||
Edit by Laura: As a biochemistry major, it is important for me to understand the interactions between molecules. Whether I am in the analytical laboratory, running mass spectrometry on a sample and need to choose whether to detect negative or positive ions, or I am in the biochemistry laboratory, running gel electrophoresis on a sample of DNA, watching the negative strands move towards the positive electrode and the positive components move towards the negative electrode, the basic principle is the same: CHARGES ARE IMPORTANT. Charges and the interactions between charged molecules are essential for chemistry, for physics, and for, in truth, life as we know it. | |||
Edit by Samyukta: As a biomedical engineering major, understanding polarization, electricity, and signaling plays a major role in not only the accurate collection of data from various medical devices and sensors that are then used to inform the development of treatments and additional products but also inherently, as understanding polarization and electricity enables me to become a more informed and aware engineer who can contribute skills beyond the otherwise somewhat isolated sphere of the lab. | |||
[[File:Microarray Comparative Genomic Hybridisation.jpg|Microarray_Comparative_Genomic_Hybridisation]] | |||
Is there an interesting industrial application? | |||
<br> | |||
The concept of polarization itself has many industrial applications. It is seen in 3D Glasses, Infrared spectroscopy, polarized sunglasses, FM radios, and even laptop screens. There are many, many industrial applications. | |||
Edit by Laura: As stated above, charges and the interactions between charged molecules are essential principles in chemistry. Electrophoresis is the process of separating a mixture using electricity. Charges flow through a sample causing the components to separate based on their charge: positively charged components are attracted to the negative electrode and negatively charged components are attracted to the positive electrode. Gel electrophoresis has played a key role in the development of vaccines and modern medicines. Additionally, it is solely responsible for the separation of DNA: a key tool in forensic identification and genetic testing. Much of modern medicine has come about simply based on the notion of charge and the interaction between positively and negatively charged species. | |||
<br> | |||
The basic set up of a gel electrophoresis experiment to separate DNA. | |||
[[File:electrode.jpg]] | |||
<br>Photo copied from Encyclopedia Britannica. | |||
<br> | |||
Edit by Samyukta: Polarization is a major fundamental concept of physics that enables a special form of imaging called tissue polarimetry in biomedical engineering (and specifically, tissue engineering). Tissue polarimetry is commonly used when the contrast and resolution of an image need to be maximal without sacrificing the integrity of the sample. The effectiveness of tissue polarimetry relies on the functionality of the polarimeter | |||
==History== | ==History== | ||
The microscopic process of polarization has expanded into the macroscopic world through light, radio waves, and industry. The macroscopic application of the microscopic phenomenon was first discovered by Etienne Louis Malus, a French physicist in the early 1800s. Malus understood that light consists of electromagnetic waves, and therefore, contains a range of radiation. The human eye is unable to see all of the waves in the range of light, but Malus used instruments and science to explore light outside of the visible spectrum, the region capable of detection by the human eye. Through his studies, Malus was able to not only determine the other frequencies of light that we cannot see, but was also able to lay the foundation for scientists to take advantage of the electromagnetic nature of light and apply the process of polarization to create today's modern technological advancements. | |||
[[File:Laser frequency comb installed on HARPS.jpg|Laser_frequency_comb_installed_on_HARPS|200px|Image: 200 pixels]] | |||
== See also == | == See also == | ||
[https://www.physicsbook.gatech.edu/Electric_Field/ Electric Field] <br> | |||
Electric Field. Electric Force. Charge Density. | [https://www.physicsbook.gatech.edu/Electric_Force/ Electric Force] <br> | ||
[https://www.physicsbook.gatech.edu/Charge_Density/ Charge Density] <br> | |||
[https://en.wikipedia.org/wiki/Static_electricity/ Static Electricity] <br> | |||
[https://en.wikipedia.org/wiki/Gel_electrophoresis/ Gel Electrophoresis] <br> | |||
===Further reading=== | ===Further reading=== | ||
Line 80: | Line 285: | ||
===External links=== | ===External links=== | ||
http://www.physicsclassroom.com/class/estatics/Lesson-1/Polarization | *http://www.physicsclassroom.com/class/estatics/Lesson-1/Polarization | ||
http://www.britannica.com/science/electric-polarization | *http://www.britannica.com/science/electric-polarization | ||
https://www.youtube.com/watch?v=HKgOpmX-OFI | *https://www.youtube.com/watch?v=HKgOpmX-OFI | ||
https://www.boundless.com/physics/textbooks/boundless-physics-textbook/electric-charge-and-field-17/overview-133/polarization-477-6289/ | *https://www.boundless.com/physics/textbooks/boundless-physics-textbook/electric-charge-and-field-17/overview-133/polarization-477-6289/ | ||
*https://www.youtube.com/watch?v=5O0yWvQhWkU | |||
*https://www.youtube.com/watch?v=W1KEgBdatN8 | |||
==References== | ==References== | ||
http://www.physicsclassroom.com/class/estatics/Lesson-1/Polarization | *http://www.physicsclassroom.com/class/estatics/Lesson-1/Polarization | ||
https://arago.elte.hu/sites/default/files/DSc-Thesis-2003-GaborHorvath-01.pdf | *https://arago.elte.hu/sites/default/files/DSc-Thesis-2003-GaborHorvath-01.pdf | ||
http://www.britannica.com/science/electric-polarization | *http://www.britannica.com/science/electric-polarization | ||
http://www.innovateus.net/science/what-polarization | *http://www.innovateus.net/science/what-polarization | ||
Matter and Interactions Volume II. | *http://www.physicsclassroom.com/class/estatics/Lesson-1/Charge-Interactions | ||
*Matter and Interactions Volume II. | |||
*https://www.youtube.com/watch?v=HKgOpmX-OFI | |||
*http://www.physicsclassroom.com/class/light/Lesson-1/Polarization | |||
http://www. | *http://www.britannica.com/science/gel-electrophoresis | ||
'''Unless otherwise stated, images were made by the author or editor.''' |
Latest revision as of 16:00, 23 April 2024
Edited By: siyer300 - Samyukta Iyer, Spring 2023
Naeha Nayar - Spring 2024
In essence, polarization describes how charged objects interact with each other in terms of the separation of charges within such objects. This article refers to electric polarization. For magnetic polarization, check Magnetic Dipole
Definitions, at a Glance
Polarization is defined theoretically defined as "the process of separating opposite charges within an object" by the application of an external electric field. Polarization is the separation of the center of positive charge and the center of negative charge in a material.
Let us first consider the case of an atom (an electric dipole) being exposed to an external electric field.
Essentially, exposure of an atom to an external electric field causes the electron cloud of an atom to surround the positively charged nucleus of the atom opposite the direction of the electric field, causing one side of the atom to be positively charged and one side to be negatively charged.
As you know, negative and positive will always repel each other. So when the positive and negative charges within an object have become separated, the object is said to be polarized.
Due to the physical separation of its positive and negative charges, a polarized object can behave like a dipole, so long as the charges remain separated. Once the electric field causing the polarization is removed and the positive and negative charges are no longer separated, the dipole disappears.
Diagram
This is also explained succinctly in the diagram below:
Because of this phenomenon, polarization is said to create induced dipoles. Error creating thumbnail: sh: /usr/bin/convert: No such file or directory Error code: 127
Polarizability
Given the phenomenon of polarization, we can then define polarizability as the ease with which the charges in an object can be separated. It measures the tendency of matter to acquire an electric dipole when it is affected by an electric field. Polarizability is a constant value, represented by the Greek character alpha, that is inherent and unique to each material and is commonly determined experimentally. It is measured in units of volume such as cubic meters. Polarizability influences a material's ability to store electrical energy when subjected to an electric field, which is important in dielectric materials used in capacitors and insulators. Molecules with more loosely held electrons or that have larger electron clouds tend to have higher polarizabilities.
Polarizability affects the strength of van der Waals forces and London dispersion forces between molecules, which influence properties such as boiling points, melting points, and solubility. Polarizability also determines how a material interacts with light, affecting the ability to scatter or absorb light.
Dipole Moment, in General
The amount of polarization an object experiences, also known as the dipole moment, is equal to the polarizability of the object multiplied by the magnitude of the applied electric field.
[math]\displaystyle{ µ = α * E }[/math]
Where µ represents the dipole moment, α represents the polarizability of the given object, and E represents the applied electric field's magnitude
- note that E and mu have a direct relationship so as the electric field's magnitude increases, the dipole moment will increase as well.
Polarization in Insulators vs. Conductors
Insulators: An insulator is a material through which mobile charges cannot flow, and a conductor is a material through which mobile charges can flow.
In an insulator, the separation of charge simply causes the electrons of the object being polarized to position themselves on the outer surfaces of the object.
It contributes to the material's dielectric properties, affecting its ability to store electrical energy when it comes into contact with an electric field.
Polarization can lead to the formation of surface charges at the interfaces between the insulator and its surroundings, which can influence the material's behavior in various applications.
In ferroelectric materials, polarization can be permanent and exhibit hysteresis behavior, meaning that the material retains its polarization even when the external electric field is removed.
Conductors: Conductors are materials characterized by their high electrical conductivity, meaning that they have free electrons that can move easily through the material in response to an applied electric field. In conductors, the electron field induces a flow within the conductor of electrons from the heavily negatively charged side of the object to the heavily positively charged side of the object. When a conductor comes into contact with an external electric field, the free electrons redistribute themselves almost instantaneously to cancel out the electric field within the conductor. This redistribution of charges leads to an equilibrium state where the net electric field inside the conductor is zero.
Clarification
It is important to note that the process of polarization in and of itself does not induce charging. Polarization is the redistribution of charges throughout an object; a polarized neutral object is still a neutral object regardless of whether it is an insulator or conductor.
Simple schematic of a polarized molecule, or a dipole
The Main Idea
At the atomic level, the application of external charges causes the subatomic particles, namely, positively-charged protons and negatively-charged electrons, to reorient with respect to the applied charge.
The external application of a positive charge on the left side of a molecule will result in the electrons of the molecule moving to the left, as they are attracted to the positive charge, and the protons stay to the right, away from the applied charge, as they repel against the positive charge.
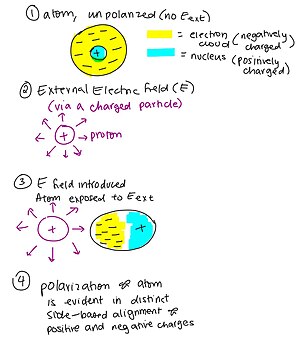
Similarly, the external application of a negative charge on the right side of a molecule will result in the protons of the molecule staying to the right, close to the applied charge, as they are attracted to the negative charge, and the electrons of the molecule move to the left, away from the applied charge, as they are repelled by the negative charge. (See Charge Interaction)
Connection between Electric Fields and Induced Dipoles
These externally applied charges, as described earlier, are electric fields. Positive electric fields point outwards, away from the center of the field, also known as its source. Negative charges, on the other hand, create inward-pointing electric fields that point toward the source of the field. The external application of a positive electric field to an object will create an outward field, resulting in the movement of the electrons of a molecule closer to the external positive charge.
The positively-charged nucleus, while it does repel against the external positive charge, cannot move around the molecule however as that would change the molecule itself. With the charges physically separated in space, an induced dipole forms. When the external positive charge is removed, the induced dipole disappears and the object is once again neutral.
On the other hand, the external application of a negative electric field to an object will create an inward field, resulting in the movement of the electrons of a molecule farther away from the external negative charge. The positively-charged nucleus will be attracted to the negative charge, but as described before, because it cannot move around the molecule, will not be pulled closer to the negative charge.
Permanent vs Induced Dipoles
Both of these instances describe scenarios drastically different from permanent dipoles, in which the positive and negative charges are always physically separated. Polarization and the resulting creation of induced dipoles help explain the seemingly magical attraction between charged objects and neutral objects.

A charged object produces an electric field that, when brought near neutral objects, generates induced dipoles. The protons and electrons reorient themselves in the presence of the charged object's electric field and attractive forces can be observed between the two objects.
Polarization in Insulators
Insulators are materials in which the electrons are tightly bound to the atoms, preventing the movement of charged particles throughout the material. As a result, electricity, which is the movement of charges, is unable to flow through the object and it is said to "insulate" one object from another. Common examples of insulators include wood, rubber, paper, and glass.
Insulators have very low polarizability constants. I.e., insulators do not polarize easily. In the presence of an externally applied electric field, the electrons in an atom shift positions slightly, but are unable to move and become separated from the protons. At most, the electrons can shift one atomic diameter or 1x10^-10 meters, but they ultimately remain attached to the atoms.
This results in the induced polarization of the individual molecules inside the insulator, as the applied electric field has caused the normally neutral object to become polarized, as opposed to entire charges moving across the material independent of their molecules (as would occur in a conductor). Within each of the individual molecules making up the insulator, dipoles have formed, but the molecules themselves have not moved. This is commonly described as the "pill" model, where each now-polarized molecule resembles a pill, with one side being positively charged and the other being negatively charged.
The magnitude of the induced polarization is dependent upon the strength of the applied electric field. The stronger the applied electric field, the greater effect it has on the insulator.
Although the molecules are not shifting, induced polarization still creates a large effect as there are many molecules to be polarized and therefore, many induced dipoles to form. In other words, the effect of the induced polarization in the insulator is felt due to the sheer quantity of slightly polarized molecules in the entire object. The separation of the positive and negative charges (polarization) is proportional to the strength of the external electric field. If the applied electric field is large enough, the induced dipoles will generate their own electric field as illustrated below.
Polarization in Conductors
Conductors are materials in which charged particles are able to flow freely, unlike insulators in which charged particles are tightly bound to the atoms.
Charged particles within conductors are able to move great distances, and their movement is also the fundamental concept enabling electricity. Common examples of conductors include silver, gold, salt water, concrete, and aluminum.
As a result of the unrestricted freedom of charged particles, polarization in conductors differs from polarization in insulators. While the electrons in an insulator are capable of reorienting themselves, the electrons in conductors can move great distances, spreading across the entire surface in response to the application of external charge.
The mobile charges can even accumulate on the outer surface of a conductor! A good example of this is Faraday's cage. A Faraday cage is essentially a hollow conductor which allows charges to accumulate on the outer surface of the cage.
After an electric field is applied to a Faraday cage, the charges on the outer surface cancel out with the charges on the inside of the cage, resulting in an equilibrium state for the cage.
Conductors in Equilibrium and Drift Speed
The process of polarization may cause the mobile charges to reorient on the surface in such a way that the net electric field goes to zero as the electric field on the surface cancels out the applied electric field. When this happens, the object is said to be in equilibrium and the electrons are no longer capable of moving through the object.
The speed with which the mobile charges move due to an applied electric field is known formally as drift speed. The drift speed is equal to the net electric field at the location of the charge multiplied by the mobility of the mobile charges. As made evident by this equation, when the object is in equilibrium, the charges stop moving.
Static Electricity
Static electricity is energy that builds up due to the interaction between charged objects. Simply put, static electricity is an imbalance between positive and negative charges. Because objects are polarizable, whether completely (conductors), or incompletely (insulators), when charged objects come into contact with each other, the electrons and protons within the object reorient or shift. As illustrated above, opposite charges attract, meaning negatively charged objects will experience an attractive force towards positively charged objects. On the other hand, similarly charged species will repel each other. When similarly charged objects are brought into contact with one another, the mobile particles will attempt to escape as quickly as possible. This rapid movement of similarly charged particles is known as static shock. Homeowners frequently experience static shock as they walk across a bedroom carpet and build up electrons on their bodies. When they reach for a doorknob or light switch, they experience static shock as the electrons quickly "escape," or discharge.
A Mathematical Model
Useful formulas for calculating polarization and its effects:
Electric Force: [math]\displaystyle{ \vec{F} = q\vec{E} }[/math] Where "F" is the applied electric force, "q" is the charge of the object being observed, and "E" is the electric field of the object.
Dipole Moment: [math]\displaystyle{ \vec{P} = \alpha \vec{E} }[/math] Where "P" is the dipole moment of the object being observed, alpha is the polarizability constant (different for every material) of the object, and "E" is the applied electric field.
Drift Speed: [math]\displaystyle{ \vec{v} = \mu E_{net} }[/math] Where "v" is the drift speed of the charged particle, mu is the mobility of the charge, and "Enet" is the magnitude of the net electric field.
A Computational Model
Use the link below to access a computational model of polarization! https://www.glowscript.org/#/user/samyukta/folder/MyPrograms/program/wiki
Examples
Simple
Determine if the statements below are True or False:
1. Charged particles can flow freely within conductors.
Click for Solution
True. There are mobile charges in conductors. Insulators do not have mobile charges as the electrons are bound tightly to the atoms.
Here is a diagram illustrating how charges move in conductors.
By contrast, here is a diagram showing how the electrons are bound securely and therefore cannot move around between atoms when in an insulator.
2. The net electric field is equal to 0 when both insulators and conductors are in equilibrium.
Click for Solution
False. The net electric field is 0 only when conductors are in equilibrium. Insulators are not able to reach equilibrium.
3. Excess charges become localized on the surface of insulators, but not on the surface of conductors.
Click for Solution
4. The average drift speed of a mobile charge is proportional to the magnitude of the net electric field of the material.
Click for Solution
True. The formula for drift speed - [math]\displaystyle{ \vec{v} = \mu E_{net} }[/math] - shows a clear proportionality between the mobility of the charge and the magnitude of the net electric field at the location of the mobile charge. Essentially, if the drift speed of a mobile charge increases, the magnitude of the net electric field of the material will also increase, and vice versa. Similarly, if the drift speed of a mobile charge decreases, the net electric field of the material will also decrease, and vice versa.

Middling
Does a negatively charged rod cause a neutral metal sphere to polarize? If so, show the polarization of the neutral metal sphere, describe the electric field (its magnitude and direction), and electric force caused by the negatively charged rod displayed below.
Click for Solution
The negative electric field points towards the negatively charged rod. The electric force is also pointed towards the charged rod. Thus, the negative mobile charges are pushed to the surface of the far right side of the sphere, away from the negatively charged rod, and the positive charges remain on the left, close to the negatively charged rod. This polarization creates a large dipole which has a zero net electric field inside the sphere.
Difficult
Find and show the polarization of Block B and Sphere C if Sphere A is a plastic sphere with a positive charge. Block B is neutral metal block while Sphere C is plastic sphere.
Click for Solution
The positive charge on Sphere A creates an electric force which repels the positive mobile charges on the block away from the positive charge while attracting negative surface charges on the block. Due to the electric force, the block polarizes in the manner illustrated below: negative charges move to the left, positive charges stay to the right. The positive surface charges on the block near sphere C cause induced polarization, forming induced dipoles within the sphere, as Sphere C is an insulator. If Sphere C were a conductor, the sphere would polarize on the outer surface, but as it stands, a mere reorientation takes places. The negative charges of Sphere C orient close to the positive surface charges of Block B.
Connectedness
How is this topic connected to something that you are interested in?
Polarization helps explain the mystical, yet fascinating, phenomena of attraction between neutral and charged objects. Polarization is evident in our everyday lives in many ways. For example, the build up of static charge on your socks as you walk across the carpet and the shock you feel when you touch a door handle as your body discharges. As with the entire field of physics, polarization helps explain the science behind many of the phenomena we experience daily that go unnoticed.
How is it connected to your major?
I am a computer engineering major. Honestly, I am not sure yet what Computer Engineering is all about. However, I am aware that polarization is an important concept in electrical engineering. Polarization of light waves seems to be more connected to my major.
Edit by Jerrin: As an electrical engineer, polarization is important in understanding electron flow in wires and other conductors and the effect of electric fields of charges on external power sources such as in the hardware of a computer or other electronics.
Edit by Laura: As a biochemistry major, it is important for me to understand the interactions between molecules. Whether I am in the analytical laboratory, running mass spectrometry on a sample and need to choose whether to detect negative or positive ions, or I am in the biochemistry laboratory, running gel electrophoresis on a sample of DNA, watching the negative strands move towards the positive electrode and the positive components move towards the negative electrode, the basic principle is the same: CHARGES ARE IMPORTANT. Charges and the interactions between charged molecules are essential for chemistry, for physics, and for, in truth, life as we know it.
Edit by Samyukta: As a biomedical engineering major, understanding polarization, electricity, and signaling plays a major role in not only the accurate collection of data from various medical devices and sensors that are then used to inform the development of treatments and additional products but also inherently, as understanding polarization and electricity enables me to become a more informed and aware engineer who can contribute skills beyond the otherwise somewhat isolated sphere of the lab.
Is there an interesting industrial application?
The concept of polarization itself has many industrial applications. It is seen in 3D Glasses, Infrared spectroscopy, polarized sunglasses, FM radios, and even laptop screens. There are many, many industrial applications.
Edit by Laura: As stated above, charges and the interactions between charged molecules are essential principles in chemistry. Electrophoresis is the process of separating a mixture using electricity. Charges flow through a sample causing the components to separate based on their charge: positively charged components are attracted to the negative electrode and negatively charged components are attracted to the positive electrode. Gel electrophoresis has played a key role in the development of vaccines and modern medicines. Additionally, it is solely responsible for the separation of DNA: a key tool in forensic identification and genetic testing. Much of modern medicine has come about simply based on the notion of charge and the interaction between positively and negatively charged species.
The basic set up of a gel electrophoresis experiment to separate DNA.
Photo copied from Encyclopedia Britannica.
Edit by Samyukta: Polarization is a major fundamental concept of physics that enables a special form of imaging called tissue polarimetry in biomedical engineering (and specifically, tissue engineering). Tissue polarimetry is commonly used when the contrast and resolution of an image need to be maximal without sacrificing the integrity of the sample. The effectiveness of tissue polarimetry relies on the functionality of the polarimeter
History
The microscopic process of polarization has expanded into the macroscopic world through light, radio waves, and industry. The macroscopic application of the microscopic phenomenon was first discovered by Etienne Louis Malus, a French physicist in the early 1800s. Malus understood that light consists of electromagnetic waves, and therefore, contains a range of radiation. The human eye is unable to see all of the waves in the range of light, but Malus used instruments and science to explore light outside of the visible spectrum, the region capable of detection by the human eye. Through his studies, Malus was able to not only determine the other frequencies of light that we cannot see, but was also able to lay the foundation for scientists to take advantage of the electromagnetic nature of light and apply the process of polarization to create today's modern technological advancements.
See also
Electric Field
Electric Force
Charge Density
Static Electricity
Gel Electrophoresis
Further reading
Matter and Interactions Volume II.
External links
References
- Matter and Interactions Volume II.
Unless otherwise stated, images were made by the author or editor.